How do individual cells of multi-cellular organisms utilize a discrete set of genetic instructions to enable cell function? Each cell in a multi-cellular organism contains essentially the same genome, encoding equivalent genetic information. Yet only a subset of the genes, the genetic instructions, is used, or expressed, in any given cell at a specified time. Regulation of spatial and temporal patterns of gene expression is central to every aspect of organismal development and normal physiological function.
Gene expression is regulated in part by the interactions of genomic DNA with packaging proteins called histones. Together, DNA wound around histones, like thread around a spool, is called chromatin. Structural constraints on DNA imposed by the packaging histone proteins can be altered by a variety of chemical modifications to the evolutionarily conserved histone proteins, including the addition of acetyl groups and methyl groups. The addition and removal of these small chemical moieties is controlled by multi-subunit molecular machines called histone modification complexes. Disruption of the balance between modified and unmodified histone states by treatment with chemical enzyme inhibitors or mutation results in loss of cell division, differentiation and/or cell death.
Toward the overarching goal of understanding how genome packaging affects gene expression to affect cell viability, division and organismal development, research in the Pile laboratory is focused on an essential histone modification complex called the SIN3 complex. We are taking a multi-pronged approach to address questions of gene regulation in the model organism Drosophila melanogaster and human lung cancer samples. Drosophila are a powerful model for these analyses as they express highly similar versions of components of the SIN3 complexes to those found in mammals, suggesting conservation of function. We utilize a combination of cellular, biochemical, molecular and genetic techniques to understand the mechanism of SIN3 gene regulation.
Current projects include:
Deciphering the response of the SIN3 proteome to environmental signals
SIN3 proteins in organisms from yeast to mammals serve as a scaffolding protein for the formation of histone deacetylase and histone demethylase complexes. Different isoforms of SIN3 associate with unique sets of proteins. Additionally, different cell types have distinct SIN3 complexes. We are currently conducting proteomic analysis to decipher the SIN3 interactome in cell types with distinct metabolic demands. Furthermore, we are analyzing the impact of oxidative stress on the role of the SIN3 isoforms in gene regulation. We are also studying how the accessory factors affect the histone modification activity of the complex to impact gene regulatory activity. We predict that SIN3 isoforms sense metabolic and stress signals, and control expression of key enzymes to promote healthy cell function.
Determining the mechanism of soft repression
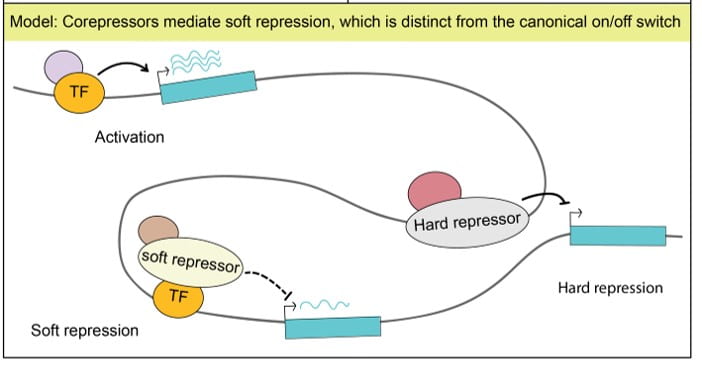
Through the course of our studies, we determined that SIN3 does not control an on/off state of gene expression for a few genes controlling cell proliferation or a specific developmental process. Instead, we find that this factor fine-tunes expression of genes encoding many key metabolic enzymes. We hypothesize that metabolic pathways, ubiquitously needed for essential cell functions, are subject to what we term “soft repression,” a modulation of gene activity, to titrate levels needed depending on cellular metabolic status. The molecular mechanisms through which the complex is able to fine-tune gene activity dependent on cellular metabolic needs is a major focus of our current research.
SIN3, metabolism and lung cancer
SIN3 controls energy production pathways and also is needed for cell proliferation. Cancer cells, which have unregulated cell division, also have rewired energy metabolism. Inhibitors of histone modifications are under consideration as anti-tumor agents as treatment of many different cancer cell lines results in loss of cell proliferation. Based on our data generated in the Drosophila model, we hypothesize that the SIN3 complex is needed for the metabolic rewiring of cancer cells. We are currently analyzing the SIN3-controlled metabolome in lung cancer cells. We are investigating the contribution of SIN3-controlled metabolites or pathways to lung cancer growth.